Zerovalent Iron (ZVI) (Chemical Reduction - ISCR)
Zerovalent iron (ZVI) is the most commonly used zerovalent metal (ZVM) for environmental remediation. ZVI is typically applied as a reductant and is capable of transforming (degrading) or sequestering a variety of contaminants found in groundwater and soil.
Related Article(s):
CONTRIBUTOR(S): Dr. Alexandra Salter-Blanc
Key Resource(s):
- Permeable Reactive Barriers of Iron and Other Zero-Valent Metals[1]
- Nanoscale Zerovalent Iron and Bimetallic Particles For Contaminated Site Remediation[2]
- The Use of Zero-Valent Iron For Groundwater Remediation and Watewater Treatment: A Review[3]
Introduction
ZVI is a reductant used in the treatment of organic and inorganic contaminants found in various environmental media, including groundwater and soil. ZVI has been shown to treat a wide variety of contaminants including chlorinated solvents, metals and metalloids, nitroaromatic compounds (including energetic compounds), nitrate, and certain dyes[1][4][2][3]. ZVI may be applied in both ex situ and in situ applications. When applied in situ, it is one of many in situ chemical reduction (ISCR) approaches. Below, we present an overview of basic ZVI redox chemistry, types of ZVI, applications, and contaminant treatment examples.
Chemistry
ZVI behaves as a reductant in many environmentally-relevant oxidation-reduction (redox) reactions. ZVI, which has a negative reduction potential, has the tendency to donate electrons (i.e., to act as a reductant and in turn be oxidized) in the presence of species with more positive reduction potentials. The redox half reaction that describes the oxidation of ZVI is:
ZVI can participate in redox reactions with a number of oxidants. For example, ZVI can be oxidized by oxygen in the presence of water to form various iron (II) species. A common example is the rusting of iron over time when oxygen and water are present. The overall redox reaction for the oxidation of ZVI in water in the presence of dissolved oxygen (DO) is given below[1]:
ZVI can also be oxidized in water in the absence of DO, although this reaction is much slower[1]:
In addition to oxygen and water, other oxidants, including certain contaminants, may also participate in redox reactions with ZVI. These reactions may result in the transformation (degradation) or immobilization of contaminants (see Contaminants Treated section below). The oxidation of ZVI also leads to the formation of various iron oxide species on the surface of the material, which can potentially adsorb contaminants (e.g., metal cations like Zn2+)[1].
Types
Many forms of ZVI are currently used in remediation applications. These include:
- Granular ZVI. Granular ZVI is used in remediation applications and is typically construction- or industrial-grade material. Sources of granular ZVI include scrap iron (e.g., from the manufacture of automotive parts), which is milled down and sorted to specific grain sizes, as well as direct production of granular material from molten iron[5].
- Nano-scale ZVI (nZVI). Nano-scale ZVI is typically defined as ZVI particles with a diameter of 1 to 100 nanometers[6]. nZVI typically has a greater reactivity than granular ZVI per mass of material. This is attributed to larger overall surface area of the nZVI particles[7].
- Bimetallic nZVI. ZVI-based bimetallic particles are composed of ZVI with a second zerovalent metal deposited on the particle surface. Bimetallic particles of ZVI doped with palladium, platinum, nickel, silver, or copper have increased reactivity toward a number of contaminants when compared to ZVI alone[2][3].
- Combination products. ZVI and nZVI are often combined with other amendments to capitalize on other remediation processes such as in situ bioremediation and sorption. For combined ZVI treatment and in situ bioremediation, biostimulants (carbon sources) are often combined with ZVI[8]. ZVI has also been combined with granular activated carbon with the intention of promoting reduction of sorbed contaminants[9]. Several commercial formulations are available for both combined approaches.
Properties and reactivity of these various forms of ZVI can vary due to the presence of “impurities” (inherent or intentionally-added) in the materials; because of this, ZVI products that fall into the same category described above may behave differently in remediation applications. Systematic research into the effects of impurities is limited.
Applications
ZVI is applied in a number of remediation applications such as:
- Permeable reactive barriers (PRBs)[1][5]
- In situ soil mixing[8]
- Injection-based treatments, including establishment of reactive treatment zones and source zone targeted injection[7][10][8]
Contaminants Treated
ZVI has been studied and applied for the treatment of a number of contaminants. The processes responsible for contaminant removal can be grouped into two main categories[1]:
- Transformation. Transformation processes involve chemical reactions that convert a contaminant to distinct products.
- Sequestration. Sequestration processes do not involve contaminant degradation, but instead primarily involve adsorption, reduction, and co-precipitation.
Here, we introduce reaction processes for some common families of contaminants:
Chlorinated solvents. Many chlorinated compounds, including the chlorinated solvents tetrachloroethene (PCE), trichloroethene (TCE), and carbon tetrachloride, are degraded by ZVI in transformation reactions known as dechlorination reactions[11][12][3][2]. The dechlorination process is a redox reaction in which ZVI serves as the reductant and the chlorinated solvent is the oxidant. The two major relevant dechlorination pathways are hydrogenolysis and reductive β-elimination[12][11] [13][1]. In hydrogenolysis (also known as reductive dehalogenation), a chlorine (or other halogen) atom in the molecule is replaced with a hydrogen atom. An example is the reduction of PCE to TCE[13] as shown in the following redox reaction:
In reductive β-elimination (also known as vicinal dehalogenation), two chlorine atoms (or other halogens) bonded to adjacent carbon atoms are removed, leaving behind two electrons. These electrons go on to form an additional bond between the carbons (i.e., a single bond becomes a double bond or a double bond becomes a triple bond). An example is the reduction of PCE to dichloroacetylene[13] as shown here:
Heavy metals and metalloids. ZVI has been applied to treatment of arsenic and heavy metal species including chromium, lead, copper, zinc, and uranium species[3][2][6][1]. The process by which these contaminants are treated can generally be categorized as a sequestration process[1]. Sequestration of these contaminants typically involves an often complex set of processes, which may include adsorption, redox reactions, co-precipitation, and precipitation[2]. These processes result in reduced mobility of the contaminants. One common heavy metal contaminant treated with ZVI is hexavalent chromium (Cr(VI)). Cr(VI) is highly toxic and mobile[2]. The sequestration process involves reduction of Cr(VI) to Cr(III) followed by Cr(III) immobilization through precipitation as Cr(OH)3 or incorporation into an iron (hydr)oxide shell on the ZVI surface[2][1] [3].
Nitroaromatic compounds. Nitroaromatic compounds (NACs), including nitrobenzene, 2,4,6-trinitrotoluene (TNT), 2,4-dinitrotoluene, and 2,6-dinitrotoluene, can be transformed through reduction by ZVI[3]. The net reduction reaction for NACs (which is a combination of several individual reduction reactions) results in the formation of an amine, as shown below, where “Ar” represents the aromatic backbone of the molecule[1][14]:
An example is the reduction of nitrobenzene to aniline[14]:
Context and Outlook
There are interconnected relationships between environmental applications of ZVMs such as ZVI and other established and emerging environmental technologies (Fig. 1)[15]. For example, ZVI was first popularized for environmental remediation because of the overlap of ZVM with PRBs. ZVI-based PRBs are now a well-established technology and applications are numerous. However, not all applications of ZVMs are PRBs, just as not all PRBs are constructed with ZVMs (Fig. 1). There is also overlap of ZVM with the growing and diverse field of nanotechnology, including the use of nZVI. Current ZVI research focuses primarily on nZVI, particularly its synthesis, modification, enhancement, and migration in environmental media. Other areas of current and future ZVI research include examining the influence of sources and impurities on reactivity, the use of combination products, and prospects for treating emerging contaminants.
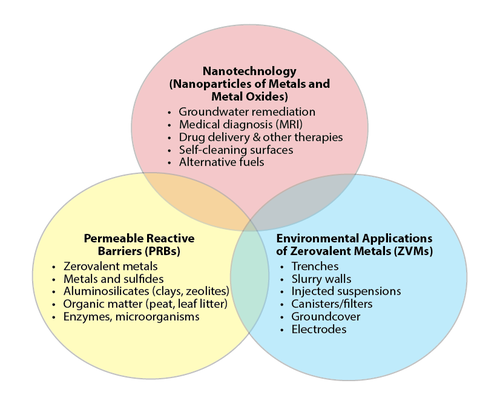
References
- ^ 1.00 1.01 1.02 1.03 1.04 1.05 1.06 1.07 1.08 1.09 1.10 1.11 Tratnyek, P.G., Scherer, M.M., Johnson, T.L. and Matheson, L.J., 2003. Permeable reactive barriers of iron and other zero-valent metals. In:Tarr MA, ed. Chemical Degradation Methods for Wastes and Pollutants: Environmental and Industrial Applications. New York, NY, Marcel Dekker, Chapter 9, pp. 371-422. doi: 10.1201/9780203912553.ch9
- ^ 2.0 2.1 2.2 2.3 2.4 2.5 2.6 2.7 O’Carroll, D., Sleep, B., Krol, M., Boparai, H. and Kocur, C., 2013. Nanoscale zero valent iron and bimetallic particles for contaminated site remediation. Advances in Water Resources, 51, pp. 104-122. doi:10.1016/j.advwatres.2012.02.005
- ^ 3.0 3.1 3.2 3.3 3.4 3.5 3.6 Fu, F., Dionysiou, D.D. and Liu, H., 2014. The use of zero-valent iron for groundwater remediation and wastewater treatment: a review. Journal of Hazardous Materials, 267, pp. 194-205. doi:10.1016/j.jhazmat.2013.12.062
- ^ Zhang, W.X., 2003. Nanoscale iron particles for environmental remediation: an overview. Journal of Nanoparticle Research, 5(3-4), pp. 323-332. doi:10.1023/A:1025520116015
- ^ 5.0 5.1 ITRC, 2011. Permeable Reactive Barrier: Technology Update. Interstate Technology and Regulatory Council. Report pdf
- ^ 6.0 6.1 Li, X.Q., Elliott, D.W. and Zhang, W.X., 2006. Zero-valent iron nanoparticles for abatement of environmental pollutants: materials and engineering aspects. Critical Reviews in Solid State and Materials Sciences, 31(4), pp. 111-122. doi:10.1080/10408430601057611
- ^ 7.0 7.1 Tratnyek, P.G. and Johnson, R.L., 2006. Nanotechnologies for environmental cleanup. Nano today, 1(2), pp. 44-48. doi:10.1016/s1748-0132(06)70048-2
- ^ 8.0 8.1 8.2 Tratnyek, P.G., Johnson, R.L., Lowry, G.V. and Brown, R.A., 2014. In situ chemical reduction for source remediation. In Chlorinated Solvent Source Zone Remediation, pp. 307-351. Springer New York. doi: 10.1007/978-1-4614-6922-3_10
- ^ Battelle, 2007. Demonstration of the AquaBlok Sediment Capping Technology: Innovative Technology Evaluation Report. U.S. Environmental Protection Agency, Washington, DC, EPA/540/R-07/008. Report pdf
- ^ CityChlor, 2013. In Situ Chemical Reduction using Zero Valent Iron Injection, April 12, 2013. Report pdf
- ^ 11.0 11.1 Roberts, A.L., Totten, L.A., Arnold, W.A., Burris, D.R. and Campbell, T.J., 1996. Reductive elimination of chlorinated ethylenes by zero-valent metals. Environmental Science & Technology, 30(8), pp. 2654-2659. doi:10.1021/es9509644
- ^ 12.0 12.1 Matheson, L.J. and Tratnyek, P.G., 1994. Reductive dehalogenation of chlorinated methanes by iron metal. Environmental Science & Technology, 28(12), pp. 2045-2053. doi:10.1021/es00061a012
- ^ 13.0 13.1 13.2 Arnold, W.A. and Roberts, A.L., 2000. Pathways and kinetics of chlorinated ethylene and chlorinated acetylene reaction with Fe (0) particles. Environmental Science & Technology, 34(9), pp. 1794-1805. doi:10.1021/es990884q
- ^ 14.0 14.1 Agrawal, A. and Tratnyek, P.G., 1995. Reduction of nitro aromatic compounds by zero-valent iron metal. Environmental Science & Technology,30(1), pp. 153-160. doi:10.1021/es950211h
- ^ 15.0 15.1 Tratnyek, P., Salter, A.J., Nurmi, J.T. and Sarathy, V., 2010. Environmental applications of zerovalent metals. Iron vs. Zinc. Nanoscale Materials in Chemistry: Environmental Applications. In American Chemical Society. Washington, DC, pp. 165-178. doi: 10.1021/bk-2010-1045.ch009
See Also
Overview of Permeable Reactive Barriers, Permeable Treatment Zones, and Application of Zero-Valent Iron Overview of In Situ Chemical Reduction
- Permeable Reactive Barriers for In-Situ Treatment of Chlorinated Solvents
- Influence of Groundwater Constituents on Longevity of Iron-Based Permeable Barriers
- Evaluation of Performance and Longevity at DoD Permeable Reactive Barrier Sites
- Remediation of Explosives Contaminated Groundwater with Zero-Valent Iron
- Fundamental Study of the Delivery of Nanoiron to DNAPL Source Zones in Naturally Heterogeneous Field Systems
- Development and Optimization of Targeted Nanoscale Iron Delivery Methods for Treatment of NAPL Source Zones
- Evaluation of Performance and Longevity at Permeable Reactive Barrier Sites
- Treatment of Chlorinated Hydrocarbon Contaminated Groundwater with Injectable Nanoscale Bimetallic Particles
- Remediation of TNT and RDX in Groundwater Using Zero-Valent Iron Permeable Reactive Barriers and Zero-Valent Iron In Situ Treatment Wells
- Emulsified Zero-Valent Nanoscale Iron Treatment of Chlorinated Solvent DNAPL Source Areas