Difference between revisions of "User:Admin/sandbox"
(Tag: Visual edit) |
|||
Line 51: | Line 51: | ||
''RDX'' | ''RDX'' | ||
− | RDX photolysis products detected in aqueous systems include [[wikipedia:Nitrite|nitrite]], [[wikipedia:Nitrate|nitrate]], [[wikipedia:Ammoni|ammonia]], [[wikipedia:Formaldehyde|formaldehyde]], [[wikipedia:Formic_acid|formic acid]], [[wikipedia:Formamide|formamide]], [[wikipedia:Nitrous_oxide|nitrous oxide]], and 4-nitro-2,4-diazabutanal<ref> | + | RDX photolysis products detected in aqueous systems include [[wikipedia:Nitrite|nitrite]], [[wikipedia:Nitrate|nitrate]], [[wikipedia:Ammoni|ammonia]], [[wikipedia:Formaldehyde|formaldehyde]], [[wikipedia:Formic_acid|formic acid]], [[wikipedia:Formamide|formamide]], [[wikipedia:Nitrous_oxide|nitrous oxide]], and 4-nitro-2,4-diazabutanal<ref>Just, C.L., and Schnoor, J.L., 2004. Phytophotolysis of Hexahydro-1,3,5-trinitro-1,3,5-triazine (RDX) in Leaves of Reed Canary Grass. Environmental Science and Technology, 38(1), pp. 290-295. [https://doi.org/10.1021/es034744z doi: 10.1021/es034744z]</ref><ref>Bordeleau, G., Martel, R., Ampleman, G., and Thiboutot, S., 2013. Photolysis of RDX and nitroglycerin in the context of military training ranges. Chemosphere, 93(1), pp. 14-19. [https://doi.org/10.1016/j.chemosphere.2013.04.048 doi: 10.1016/j.chemosphere.2013.04.048]</ref><ref>Glover, D.J., and Hoffsommer, J.C., 1979. Photolyis of RDX in Aqueous Solution, With and Without Ozone. Naval Service Weapons Center, NSWC/WOL TR 78-175. [[Media: ADA1979.pdf | Report]]</ref><ref>Hawari, J., Halasz, A., Groom, C., Deschamps S., Paquet, L., Beaulieu, C., and Corriveau, A., 2002. Photodegradation of RDX in Aqueous Aolution: A Mechanistic Probe for Biodegradation with Rhodococcus sp. Environmental Science and Technology, 36(23), pp. 5117-5123. [https://doi.org/10.1021/es0207753 doi: 10.1021/es020775]</ref>. Stable phototransformation products also detected include nitroso compounds MNX (hexahydro-1-nitroso-3,5-dinitro-1,3,5-triazine), DNX (hexahydro-1,3-dinitroso-5-nitro-1,3,5-triazine), and TNX (hexahydro-1,3,5-trinitroso-1,3,5-triazine), which contain one, two, and three nitroso groups each, respectively, in place of the nitro groups. The formation of unsaturated MUX (1,3-dinitro-1,2,3,4-tetrahydro-1,3,5-triazine) was also reported (Figure 7)<ref name=":6" />. |
− | |||
− | |||
− | Bordeleau, G., Martel, R., Ampleman, G., and Thiboutot, S., 2013. Photolysis of RDX and nitroglycerin in the context of military training ranges. Chemosphere, 93(1), pp. 14-19. [https://doi.org/10.1016/j.chemosphere.2013.04.048 doi: 10.1016/j.chemosphere.2013.04.048] | ||
− | |||
− | Glover, D.J., and Hoffsommer, J.C., 1979. Photolyis of RDX in Aqueous Solution, With and Without Ozone. Naval Service Weapons Center, NSWC/WOL TR 78-175. [[Media: ADA1979.pdf | Report]] | ||
− | |||
− | Hawari, J., Halasz, A., Groom, C., Deschamps S., Paquet, L., Beaulieu, C., and Corriveau, A., 2002. Photodegradation of RDX in Aqueous Aolution: A Mechanistic Probe for Biodegradation with Rhodococcus sp. Environmental Science and Technology, 36(23), pp. 5117-5123. [https://doi.org/10.1021/es0207753 doi: 10.1021/es020775] | ||
− | |||
<br /> | <br /> | ||
<references /> | <references /> |
Revision as of 22:17, 14 December 2021
I have installed SandboxLink extension that provides each user their own sandbox accessible through their personal menu bar (top right)
Munitions Constituents – Photolysis
Munitions compounds (MCs), including 2,4,6-trinitrotoluene (TNT), hexahydro-1,3,5-trinitro-1,3,5-triazine (RDX), 2,4-dinitroanisole (DNAN), 3-nitro-1,2,4-triazol-5-one (NTO), and nitroguanidine (NQ), absorb light in the UV range and are therefore susceptible to photolysis on soil surfaces and in surface water. Photochemical reactions are important to consider when assessing the environmental impact of MCs since they can yield products that differ from their parent compounds in both toxicity and transport behavior. Quantum yield calculations can aid in predicting the photolysis rates and half-lives of MCs. The photolysis of MCs may be enhanced or inhibited in the presence of compounds that are also excited by UV irradiation. Munitions compounds (MCs), including 2,4,6-trinitrotoluene (TNT), hexahydro-1,3,5-trinitro-1,3,5-triazine (RDX), 2,4-dinitroanisole (DNAN), 3-nitro-1,2,4-triazol-5-one (NTO), and nitroguanidine (NQ), absorb light in the UV range and are therefore susceptible to photolysis on soil surfaces and in surface water. Photochemical reactions are important to consider when assessing the environmental impact of MCs since they can yield products that differ from their parent compounds in both toxicity and transport behavior. Quantum yield calculations can aid in predicting the photolysis rates and half-lives of MCs. The photolysis of MCs may be enhanced or inhibited in the presence of compounds that are also excited by UV irradiation.
Related Article(s):
Contributor(s): Dr. Warren Kadoya
Key Resource(s):
- Environmental Organic Chemistry, Chapter 15: Direct Photolysis[1]
- Photochemical Degradation of Composition B and Its Components[2]
- Verification of RDX Photolysis Mechanism[3]
- Photochemical transformation of the insensitive munitions compound 2,4-dinitroanisole[4]
- Photo-transformation of aqueous nitroguanidine and 3-nitro-1,2,4-triazol-5-one: Emerging munitions compounds[5]
Introduction
Insensitive munitions, including IMX-101 and IMX-104, are replacing traditional explosives because they are less prone to accidental detonation and therefore safer for military personnel to handle. IMX-101, composed of 2,4-dinitroanisole (DNAN), 3-nitro-1,2,4-triazol-5-one (NTO), and nitroguanidine (NQ), will replace 2,4,6-trinitrotoluene (TNT) in artillery; IMX-104, composed of DNAN, NTO, and hexahydro-1,3,5-trinitro-1,3,5-triazine (RDX), will replace Composition B (Comp B) in mortars[6]. As both traditional munitions compounds and these insensitive munitions compounds (collectively referred to as MCs) may be deposited onto firing ranges via incomplete detonation, understanding their environmental fate is of concern[7]. Phototransformation due to sunlight exposure is an important fate-controlling parameter for MCs and can occur on the surfaces of solid explosive particles, as shown in Figure 1, as well as in the aqueous phase following MC dissolution by rainwater. Furthermore, MC photolysis can be affected by the presence of natural organic matter and other compounds that are excited by sunlight.
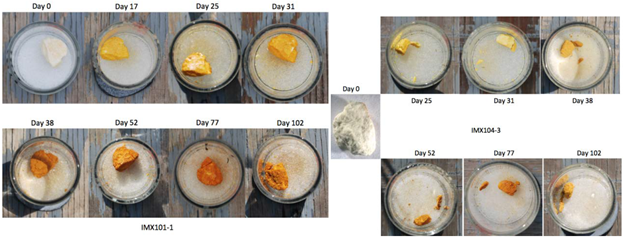
Direct photolysis
Compounds that absorb ultraviolet (UV, 100-400 nm) and/or visible (Vis, 400-800 nm) light can undergo direct photolysis from sunlight. Light is absorbed in discrete units, or photons, and the energy of these photons is indirectly proportional to the wavelength of the light. When a chemical molecule absorbs a photon, its ground state electrons may become excited. As the excited electrons return to the ground state, they may undergo a chemical reaction that results in transformation. Figure 2 shows that the UV range is further divided into UV-A (315-400 nm), UV-B (280-315 nm), and UV-C (100-280 nm). Because most of UV-C is filtered out by the Earth’s atmosphere, direct photolysis of compounds at the surface primarily involves UV-A and UV-B[9].
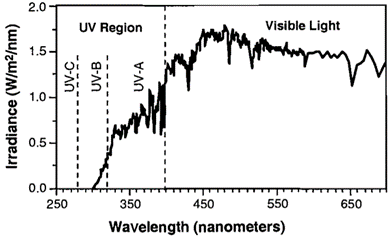
The MCs TNT, RDX, DNAN, NTO, and NQ may undergo direct photolysis since they all absorb light in the UV-Vis range (Figure 3). These graphs convey the probability that the compounds will absorb light at a given wavelength. The absorption maxima correspond to one or more electrons transitioning to an excited state.
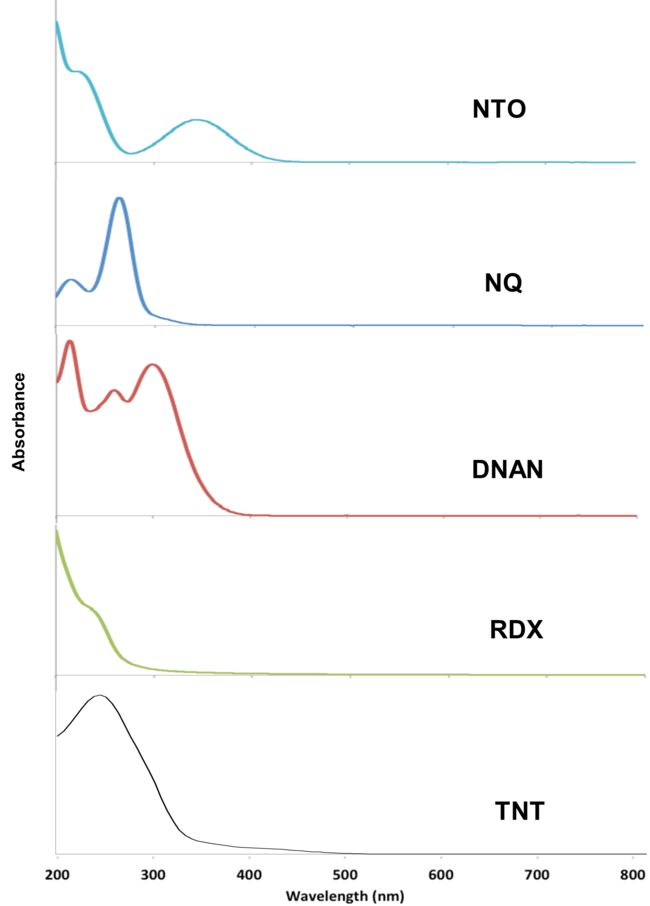
For a chemical bond to break via direct photolysis, a molecule must absorb a photon with higher energy than the energy of the bond. The energy of photons in the UV-Vis range is similar to the bond energies of several single covalent bonds found in organic molecules[1]. Therefore, many of the bonds in TNT, DNAN, NTO, and NQ are susceptible to photolysis from sunlight exposure (Figure 4).
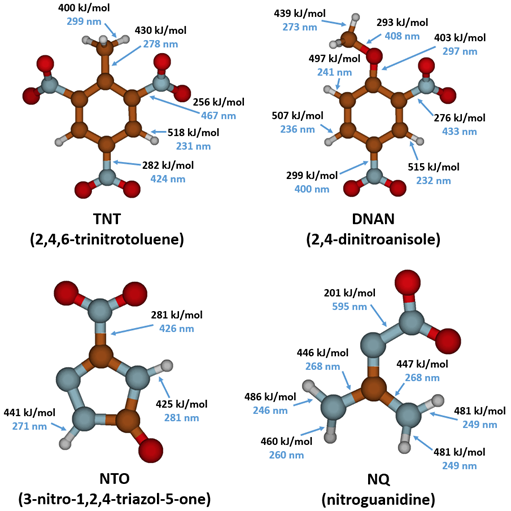
Photolysis products and pathways
The products of the direct photolysis of MCs have been studied in depth, both in photoreactors using UV bulbs emitting light at a given wavelength (Figure 5) or wavelength ranges (including simulated sunlight) and outdoors in natural sunlight. The photolysis of MCs can form mineral products as well as transformation products that may be more toxic than the original compounds.
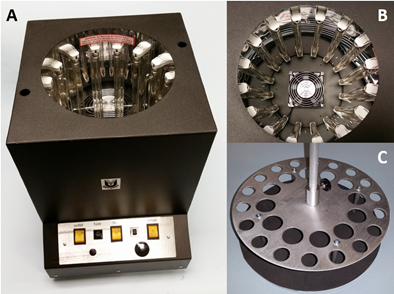
TNT
The formation of pink and red wastewater released by some ammunition plants led to investigations into the photolysis products of TNT starting in the 1970s. Laboratory experiments observed rapid phototransformation from sunlight in natural waters, some with half-lives less than an hour[12]. Numerous photolysis products of TNT have been reported and include, but are not limited to, 2-amino-4,6-dinitrobenzoic acid, 2,4,6-trinitrobenzaldehyde, 4,6-dinitroanthranil, 2,4,6-trinitrobenzonitrile, 2,4,6-trinitrobenzoic acid, 1,3,5-trinitrobenzene (TNB), 2,4,6-trinitrobenzyl alcohol, and an array of azo and azoxy compounds[2][13][14][15][16].
The mechanism of TNT photolysis is not completely understood as numerous products are formed, many of which are not readily synthesized or purchased[16]. However, evidence suggests that TNT is initially excited to a triplet state by UV light[12]. Figure 6 shows a proposed environmental reaction pathway for TNT that combines photo- and biological reactions based on studies in waste disposal lagoons[15].
The aquatic toxicity of phototransformed TNT solutions and ammunition wastewaters was not found to be significantly different from that of untransformed TNT[18][19]. Although TNT phototransformation products tend to be more toxic than TNT, the relatively low product formation coupled with the disappearance of TNT may explain these results.
RDX
RDX photolysis products detected in aqueous systems include nitrite, nitrate, ammonia, formaldehyde, formic acid, formamide, nitrous oxide, and 4-nitro-2,4-diazabutanal[20][21][22][23]. Stable phototransformation products also detected include nitroso compounds MNX (hexahydro-1-nitroso-3,5-dinitro-1,3,5-triazine), DNX (hexahydro-1,3-dinitroso-5-nitro-1,3,5-triazine), and TNX (hexahydro-1,3,5-trinitroso-1,3,5-triazine), which contain one, two, and three nitroso groups each, respectively, in place of the nitro groups. The formation of unsaturated MUX (1,3-dinitro-1,2,3,4-tetrahydro-1,3,5-triazine) was also reported (Figure 7)[3].
- ^ 1.0 1.1 1.2 Schwarzenbach, R.P., Gschwend, P.M., and Imboden, D.M., 2002. Chapter 15, Direct Photolysis. In: Schwarzenbach, R.P., Gschwend, P.M., and Imboden, D.M. (eds). Environmental Organic Chemistry. 2nd ed. Hoboken, NJ: John Wiley & Sons, Inc, pp. 611-654. doi:10.1002/0471649643.ch15
- ^ 2.0 2.1 Pennington, J.C., Thorn, K.A., Co, L.G., MacMillan, D.K., Yost, S., and Laubscher, R.D., 2007. Photochemical Degradation of Composition B and Its Components. U.S. Army Engineer Research and Development Center (ERDC)/ Environmental Laboratory (EL) TR-07-16. Report
- ^ 3.0 3.1 Peyton, G.R., LeFaivre, M.H., and Maloney, S.W., 1999. Verification of RDX photolysis mechanism. U.S. Army Engineer Research and Development Center (ERDC)/ Construction Engineering Research Laboratory (CERL) TR 99/93. Report
- ^ Rao, B., Wang, W., Cai, Q., Anderson, T., and Gu, B., 2013. Photochemical Transformation of The Insensitive Munitions Compound 2,4-Dinitroanisole. Science of The Total Environment, 443, pp. 692-699. doi: 10.1016/j.scitotenv.2012.11.033
- ^ Becher, J.B., Beal, S.A., Taylor, S., Dontsova, K., Wilcox, D.E., 2019. Photo-transformation of aqueous nitroguanidine and 3-nitro-1,2,4-triazol-5-one: Emerging munitions compounds. Chemosphere, 228, pp. 418-426. doi:10.1016/j.chemosphere.2019.04.131
- ^ BAE Systems, 2021. Making explosives safer
- ^ Pennington, J.C., Silverblatt, B., Poe, K., Hayes, C.A., and Yost, S, 2008. Explosive residues from low-order detonations of heavy artillery and mortar rounds. Soil and Sediment Contamination: An International Journal, 17(5), pp. 533-546. doi: 10.1080/15320380802306669
- ^ Dontsova, K., Taylor S., Pesce-Rodriguez, R., Brusseau, M., Arthur, J., Mark, N., Walsh, M., Lever, J., and Simunek, J., 2014. Dissolution of NTO, DNAN, and insensitive munitions formulations and their fates in soils. U.S. Army Engineer Research and Development Center (ERDC)/ Cold Region Research and Engineering Laboratory (CRREL) TR-14-23. Report
- ^ 9.0 9.1 Brennan, P., and Fedor, C., 1994. Sunlight, UV, & accelerated weathering. Q-Lab Corporation, Technical Bulletin LU-0822. Paper
- ^ Taylor, S., Becher, J., Beal, S., Ringelberg, D., Spanggord, R., and Dontsova, K., 2017. Photo-transformation of explosives and their constituents. In: The Environmental Aspects of Munitions Workshop, Joint Army-Navy-NASA-Air Force (JANNAF), Kansas City, MO, May 22, 2017.
- ^ Dontsova, K., Taylor, S., Brusseau, M. L., Simunek, J., and Hunt, E., 2017. Influence of climate on dissolution and phototransformation of NTO and DNAN from insensitive munitions and their fate in soils. In: Poster, the SERDP-ESTCP symposium, Washington, D.C., November 28-30, 2017. The Strategic Environmental Research and Development Program (SERDP) and Environmental Security Technology Certification Program (ESTCP).
- ^ 12.0 12.1 Mabey, W.R., Tse, D., Baraze, A., and Mill, T., 1983. Photolysis of nitroaromatics in aquatic systems. I. 2,4,6-trinitrotoluene. Chemosphere, 12(1), pp. 3-16. doi: 10.1016/0045-6535(83)90174-1
- ^ Burlinson, N.E., Kaplan, L.A., and Adams, C.E., 1983. Photochemistry of TNT: Investigation of the ‘pink water’ problem. Naval Ordnance Laboratory, pp. 73-172. Report
- ^ Spanggord, R.J., Mill, T., Chou, T., Mabey, W.R., Smith, J.H., and Lee, S., 1980. Environmental fate studies on certain munition wastewater constituents. Phase II - Laboratory studies. U S Army Biomedical Research and Development Laboratory. Report
- ^ 15.0 15.1 15.2 Spanggord, R.J., Mabey, W.R., Mill, T., Tsong-Wen, C., Smith, J.H., Lee, S., and Roberts, D., 1983. Environmental fate studies on certain munitions wastewater constituents: Phase IV - Lagoon model studies. U S Army Biomedical Research and Development Laboratory. Report
- ^ 16.0 16.1 Luning Prak D.J., Breuer J.E.T., Rios E.A., Jedlicka E.E., and O'Sullivan D.W., 2017. Photolysis of 2,4,6-trinitrotoluene in seawater and estuary water: Impact of pH, temperature, salinity, and dissolved organic matter. Marine Pollution Bulletin, 114(2), pp. 977-986. doi: 10.1016/j.marpolbul.2016.10.073
- ^ Walsh, M.E., 1990. Environmental Transformation Products of Nitroaromatics and Nitramines: Literature Review and Recommendations for Analytical Method Development. US Army Corps of Engineers Cold Regions Research and Engineering Laboratory. Report
- ^ Liu, D.H.W., Spanggord, R.J., Bailey, H.C., Javitz, H.S., and Jones, D.C.L., 1983. Toxicity of TNT Wastewaters to Aquatic Organisms. Volume 1. Acute Toxicity of LAP Wastewater and 2,4,6-Trinitrotoluene. U S Army Medical Bioengineering Research and Development Laboratory. Report
- ^ Kennedy, A.J., Poda, A.R., Melby, N.L., Moores, L.C., Jordan, S.M., Gust, K.A., and Bednar, A.J., 2017. Aquatic toxicity of photo-degraded insensitive munition 101 (IMX-101) constituents. Environmental Toxicology and Chemistry, 36(8), pp.2050-2057. doi: 10.1002/etc.3732
- ^ Just, C.L., and Schnoor, J.L., 2004. Phytophotolysis of Hexahydro-1,3,5-trinitro-1,3,5-triazine (RDX) in Leaves of Reed Canary Grass. Environmental Science and Technology, 38(1), pp. 290-295. doi: 10.1021/es034744z
- ^ Bordeleau, G., Martel, R., Ampleman, G., and Thiboutot, S., 2013. Photolysis of RDX and nitroglycerin in the context of military training ranges. Chemosphere, 93(1), pp. 14-19. doi: 10.1016/j.chemosphere.2013.04.048
- ^ Glover, D.J., and Hoffsommer, J.C., 1979. Photolyis of RDX in Aqueous Solution, With and Without Ozone. Naval Service Weapons Center, NSWC/WOL TR 78-175. Report
- ^ Hawari, J., Halasz, A., Groom, C., Deschamps S., Paquet, L., Beaulieu, C., and Corriveau, A., 2002. Photodegradation of RDX in Aqueous Aolution: A Mechanistic Probe for Biodegradation with Rhodococcus sp. Environmental Science and Technology, 36(23), pp. 5117-5123. doi: 10.1021/es020775